Quantum computers have the potential to complete specific algorithms at higher speeds and greater accuracy — beyond the power of our most advanced supercomputers. To best understand quantum computing and its massive potential, we must first understand the purpose of computing itself.
Classical computing exists to process and manipulate data. These computers manipulate “bits” to store data in the binary language (a series of 1’s and 0’s). Because bits are binary, they can only exist in one state at a time — each bit can only be “1” or “0,” never both.
Similarly, quantum computers use “quantum bits” or “qubits” to store and manipulate information. Not so similarly, qubits can exist in many states simultaneously. This trait of qubits is fundamental to quantum mechanics and computing.
Benefits of Quantum Computing
Qubits take time and effort to make — unlike bits, they’re not readily accessible. This plays a huge role in the production and implementation of quantum computers. There are two primary ways to make qubits.
One way is by cooling superconducting circuits to extremely low temperatures, qubits can be isolated in a controlled quantum state.
The second way is by creating an extreme vacuum chamber, individual atoms can be trapped in electromagnetic fields on silicon chips, thus isolating qubits in a controlled quantum state.
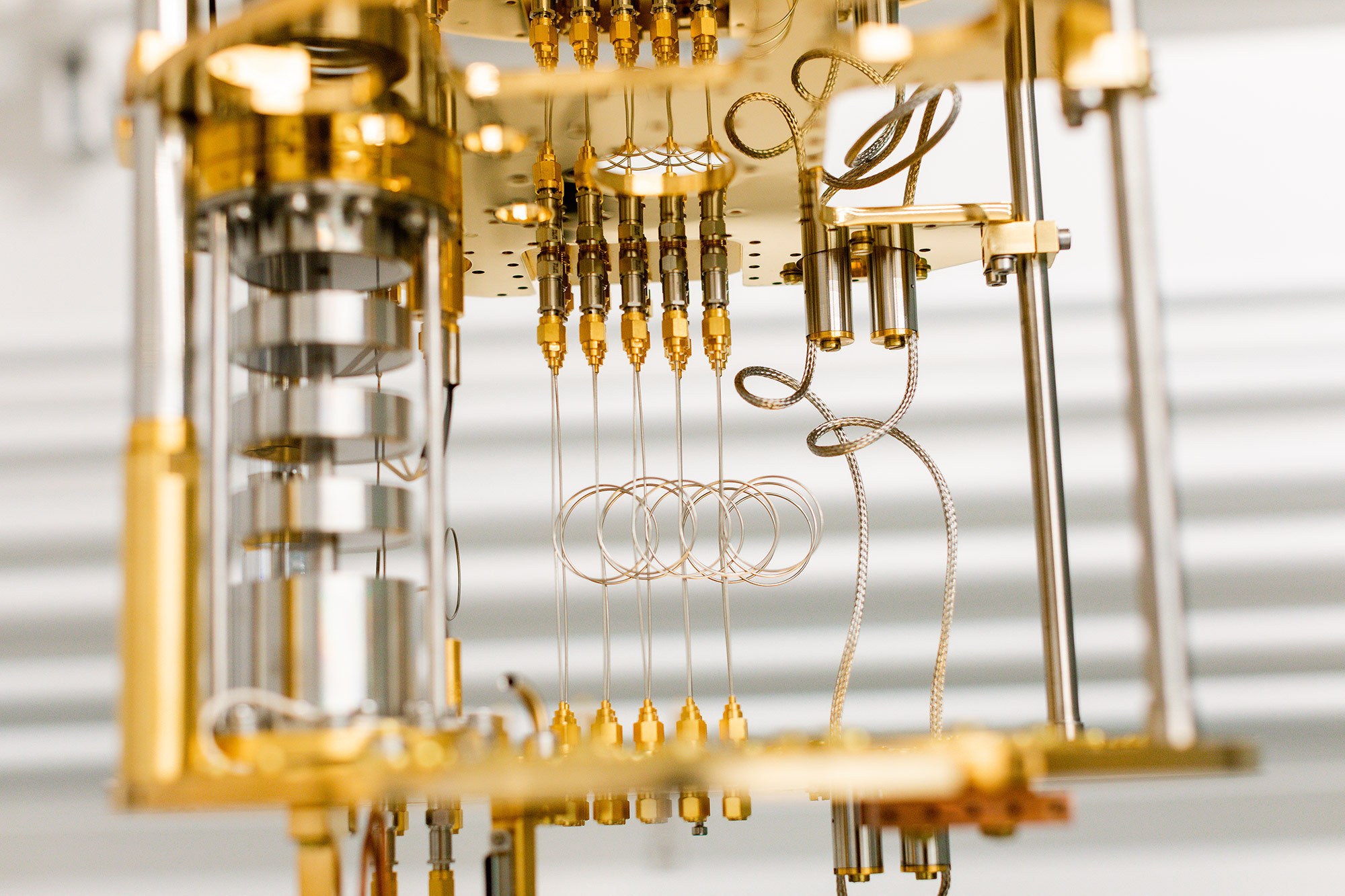
In order to control the input and output of a quantum computer, we must control the state of qubits, that is, to put them in a usable state. To best control the state of a qubit, quantum computing uses three primary mechanical traits.
Superposition
This occurs when a qubit is in a combination of states. In classical computing terms, this would be if a bit were both “1” and “0” at the same time.
Entanglement
This occurs when a pair of qubits exist in the same quantum state, working together as a system.
Interference
This occurs when qubits interact or respond to their surroundings, causing their behavior to slow and then stop. Interference allows us to manipulate the qubit to different states.
Fault Tolerance and Quantum Supremacy
Quantum computers can perform several functions and algorithms, the most common application being an algorithm that finds the “best” solution among many. Currently, most quantum computers can only run one algorithm at a time, using huge amounts of energy. The future of goal quantum computers is a fault-tolerant quantum computer, one that would be able to run several algorithms simultaneously and remain running for long periods of time, all while using less energy than existing technology.
There are two main goals when trying to achieve a fault-tolerant quantum computer: a high qubit count and a low error-rate.
With more qubits, a quantum computer can manipulate and store more data. The problem is that unlike regular bits, qubits must go through a physical process to be created and maintained, thus slowing the process of achieving a high qubit count. To accurately manipulate the necessary qubits, a low error rate is needed. This would allow the machine to provide factually accurate information instead of meaningless noise from the system.
The achievable height of quantum computing is quantum supremacy. A quantum computer has reached supremacy when it can complete an algorithm that is conclusively beyond the power of advanced supercomputers. This was achieved by Google in 2019 when Dr. John Martinis led an experiment in which a quantum computer carried out a specific calculation that is beyond the practical capabilities of regular, ‘classical’ computers.
Commercialization
While many consider quantum supremacy to be the end-all-be-all goal for quantum computing, the true test is commercialization. Quantum computers can be commercialized when application-specific quantum computing systems can be targeted to solve current, on-going problems. Without commercialization, there is little real-world incentive for quantum computing.
To reach commercialization, quantum computers must have the properties of fault tolerant systems but be scaled down to a reasonable size, structure, and use-case.
Commercialization is where SEEQC differs from its counterparts.